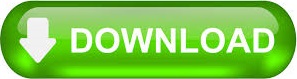
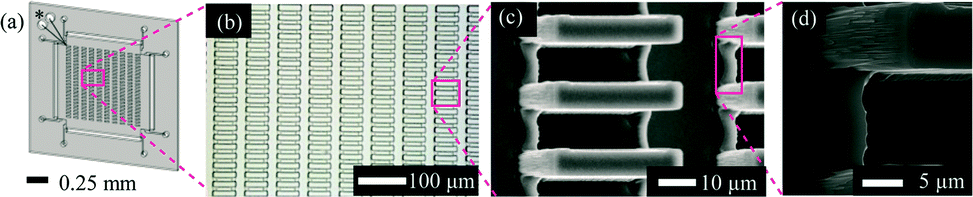
We developed a novel method for producing hydrogel microspheres, termed core-shell spherification (CSS). An excellent review of the different methods used to create microspheres from PEG or HA, including photolithography, micromolding, and emulsion, can be found in Choe et al. 17, 18 However, scaling these methods to the levels needed for transplants can be challenging. 14–16 Patterned molds are a way to circumvent the cytotoxicity issues and still create microspheres. Often, the emulsion protocols include harsh nonaqueous solvents that can lead to cytotoxicity issues or poor process control, resulting in highly variable microsphere shapes and sizes. Protocols have been developed for producing microspheres using alternative hydrogels such as polyethylene glycol (PEG), agarose, chitosan, or hyaluronic acid (HA), but are based on oil-emulsion techniques. 8 Unfortunately, these hydrogels have slower gelation times than alginate, and thus are typically prepared as macroscopic structures, 10–12 which can result in diffusion limitations due to the low surface area. In contrast, there is an abundance of alternative hydrogels that are synthetically manufactured, eliminating the variation associated with alginate purification steps. 3 Others have focused on the poor long-term biocompatibility of calcium alginate, 4–6 noting that the purification of alginate is essential to its biocompatibility. For example, the weakness of the alginate microspheres led researchers to alter the formulation for improved mechanical resistance required during injections. However, alginate does present some challenges that researchers have attempted to resolve with numerous variations in the alginate fabrication process. 1 Encapsulation with alginate has persisted as the clear material of choice for cell therapies due to alginate's unique, nearly instantaneous, crosslinking kinetics, enabling straightforward fabrication by dropping aqueous sodium alginate into a bath of crosslinking calcium. The concept of cell encapsulation was introduced in 1980 by Lim and Sun, who showed that islets embedded in alginate microspheres could reverse diabetes in rats without the need for immunosuppression, although for only a few weeks. In conclusion, CSS provides a nontoxic microencapsulation procedure compatible with various hydrogel types. Nonencapsulated canine islet transplanted at the same dose did not restore normoglycemia for any length of time. In contrast, islets encapsulated in MeHA microspheres at the same dose restored normoglycemia, but only transiently (3–4 weeks). PEGDA microspheres reversed diabetes for the length of the study (up to 16 weeks). To test the ability of the hydrogels to protect cells, while promoting function, diabetic NOD mice received intraperitoneal injections of PEGDA or MeHA microencapsulated canine islets. In contrast, PEGDA microspheres had the smallest diameters, the lowest swelling ratio, and the highest diffusion barrier, while microspheres of thiolated HA had characteristics that were in between the other two groups. Microspheres of methacrylated HA (MeHA) had the greatest swelling ratio, the largest average diameter, and the lowest diffusion barrier. HA microspheres were manufactured with two different crosslinking methods: thiolation and methacrylation. Fabrication of microspheres by CSS derived from two slow-hardening hydrogels, hyaluronic acid (HA) and polyethylene glycol diacrylate (PEGDA), was characterized.

We have developed a novel, noncytotoxic, nonemulsion-based method to produce hydrogel microspheres compatible with a wide variety of materials, called core-shell spherification (CSS). Traditional alginate microspheres suffer from poor biocompatibility, and microencapsulation of more advanced hydrogels is challenging due to their slower gelation rates. Cell microencapsulation is a rapidly expanding field with broad potential for stem cell therapies and tissue engineering research.
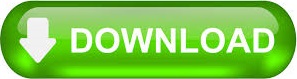